Anatomic Considerations Of Kinematics
Published .
Injury to the Head (Brain and Maxillofacial Injury)
Injury to the superficial regions of the brain is explained by these linear principles; however, injury to the deep structures of the brain, such as diffuse axonal injury (DAI), is more complicated. Several authors have tried to explain DAI as a result of shear strain between different parts of the brain, but there is also another model known as the stereotactic phenomenon. This model relies more on wave propagation and utilizes the concavity of the skull as a “collector,” which focuses multiple wave fronts to a focal point deep within the brain, causing disruption of tissue even in the face of minimal injury at the surface of the brain. This “wave propagation” through deeper structures within the brain, such as the reticular-activating system, with subsequent disruption of their structural integrity is thought to account for a loss of consciousness, the most frequent serious sign after blunt trauma to the brain. Current research characterizes DAI as a progressive process induced by the forces of injury, gradually evolving from focal axonal alteration to eventual disconnection. Traumatically induced focal axolemmal permeability leads to local influx of Ca2+ causing the release of proteases that digest the “membrane skeleton.” This ultimately leads to local axonal failure and disconnection.51 An injury caused by shear strain is a laceration or contusion of the brainstem. This is explained by opposing forces applied to the brain and the spinal cord perpendicular to their line of orientation, with the spinal cord and brainstem being relatively fixed in relation to the mobile brain.
Maxillofacial injuries are associated with injuries to the head and brain in terms of mechanism and are a common presentation after motor vehicle crashes. The classic force vector that results in mid-face fractures is similar to that of traumatic brain injury and occurs when a motor vehicle occupant impacts the steering wheel, dashboard, or windshield. Nearly all of these subtypes of injury are secondary to compressive strain. This mechanism is associated with the greatest morbidity for the driver and front-seat passenger, while the forces are attenuated for the back-seat passenger impacting the more compliant front seat.
Thoracic Injury

The primary mechanism of blunt trauma to the chest wall involves inward displacement of the body wall with impact. Musculoskeletal injury in the chest is dependent upon both the magnitude and rate of the deformation of the chest wall and is usually secondary to compressive strain from the applied load. Patterns of injury for the internal organs of the thorax frequently reflect the interactions between organs that are fixed and those that are relatively mobile and compressible. This arrangement allows for differentials in momentum between adjacent structures that lead to compressive, tensile, and shear stresses.
The sternum is deformed and rib cage compressed with a blunt force to the chest. Depending on the force and rate of impact in a collision, ribs may fracture from compressive strain applied to their outer surface and consequent tensile strain on the inner aspects of the rib. Indirect fractures may occur due to stress concentration at the lateral and posterolateral angles of the rib. Furthermore, stress waves may propagate deeper into the chest resulting in small, rapid distortions or shear forces in an organ with significant pressure differential across its parenchymal surface (i.e., the air and tissue interface of the lung). This is thought to be the mechanism causing a pulmonary contusion.
Blunt intrusion into the hemithorax and a pliable lung could also result in overpressure and cause a pneumothorax. A direct load applied to the chest compresses the lung and increases the pressure within this air-filled structure beyond the failure point of the alveoli and visceral pleura. This overpressure mechanism may also be seen with fluid (blood) instead of air in a blunt cardiac rupture. High-speed cine-radiography in an anterior blunt chest trauma model in the pig has demonstrated that the heart can be compressed to half of its pre-crash diameter with a doubling of the pressure within the cardiac chambers. If the failure point is reached, rupture occurs with disastrous results.

Abdominal Injury
Abdominal organs are more vulnerable than those of the thorax because of the lack of protection by the sternum and ribs. A number of different mechanisms account for the spectrum of injury observed in blunt trauma to the abdomen. With regard to the solid abdominal organs, a direct compressive force with parenchymal destruction probably accounts for most observed injuries to the liver, spleen, and kidney. Shear strain can also contribute to laceration of these organs. As with the previous description of strain forces, a point of attachment is required to exacerbate a differential in movement. This can occur at the splenic hilum resulting in vascular disruption at the pedicle or at the ligamentous attachments to the kidney and diaphragm. Shear forces in the liver revolve upon the attachments of the falciform ligament anteriorly and the hepatic veins posteriorly, explaining injuries to the parenchyma in these areas. Another significant injury related to this mechanism is injury to the renal artery. The renal artery is attached proximally to the abdominal aorta, which is fairly immobile secondary to its attachments to the spinal column, and distally to the kidney, which has more mobility. A discrepancy in momentum between the two will exact a shear stain on the renal artery resulting in disruption. This same relation to the spinal column occurs with the pancreas. The relatively immobile spine and freely mobile pancreatic tail predispose to a differential in momentum between the two in a deceleration situation leading to fracture in the neck or body of the pancreas. The biomechanics of such injuries suggest that the body’s tolerance to such forces decreases with a higher speed of impact, resulting in an injury of greater magnitude from a higher velocity collision.

Perforation of a hollow viscus in blunt abdominal trauma occurs in approximately 3% of victims. The exact cause is a matter of debate. Some believe that it is related to compressive forces, which cause an effective “blowout” through generation of significant overpressure, whereas others believe that it is secondary to shear strains. Both explanations are plausible, and clinical observations have supported the respective conclusions. Most injuries to the small bowel occur within 30 cm of the ligament of Treitz or the ileocecal valve, supporting the shear force theory. Yet, injuries do occur away from these points of fixation. Also, experiments have documented that a “pseudo-obstruction” or temporarily closed loop under a load can develop bursting pressures as described by the overpressure theory. Clinically, this is confirmed by the largest percentage of small intestinal injuries being of the “blowout” variety. Most likely, both proposed mechanisms are applicable in individual instances. The most common example of the pseudo-obstruction type is blunt rupture of the duodenum, where the pylorus and its retroperitoneal location can prevent adequate escape of gas and resultant high pressures that overcome wall strength.
Another important example of overpressure is rupture of the diaphragm. The peritoneal cavity is also subject to Boyle’s law, which states that volume of a gas is inversely proportional to pressure. A large blunt force, such as that related to impact with the steering wheel, applied to the anterior abdominal wall will cause a temporary deformation and decrease in the volume of the peritoneal cavity. This will subsequently raise intraabdominal pressure. The weakest point of the cavity is the diaphragm with the left side being the preferred route of pressure release as the liver absorbs pressure and protects the right hemidiaphragm. The relative deformability of the lung on the other side of the diaphragm facilitates this.
Musculoskeletal Injury
By far, the most common type of blunt injury in industrialized nations is to the musculoskeletal system. The ratio of orthopedic operations to general surgical, thoracic, and neurosurgical operations is nearly 5:1. As stated earlier, seatbelts and air bags have significantly decreased the incidence of major intracranial and abdominal injuries; however, they have not decreased the incidence of musculoskeletal trauma. Although these are not usually fatal injuries, they often require operative repair and rehabilitation and can leave a significant proportion of patients with permanent disability. With the advent of seatbelt laws, improved restraint systems, and air bags in motor vehicles, the incidence of lower extremity trauma, in particular, has increased. It is thought that these patients in the past may have suffered fatal injuries to the brain or torso and, therefore, their associated fractures of the femur, tibia, and fibula were not included in the overall list of injuries.
The type and extent of injury is determined by the momentum and kinetic energy associated with impact, underlying tissue characteristics, and angle of stress of the extremity. High-energy injuries can involve extensive loss of soft tissue, associated neurovascular compromise, and highly comminuted fracture patterns. Low-energy injuries are often associated with crush or avulsion of soft tissue in association with simple fractures. Injuries to soft tissue are usually secondary to compressive strain with crush injury as an example. Tensile and shear strain mechanisms, however, are present with degloving and avulsion injuries, respectively.
Most of that written about musculoskeletal injury involves fractures of long bones. Although each fracture is probably a consequence of multiple stresses and strains, there are four basic biomechanisms In a lateral load applied to the mid shaft of a long bone, bowing will occur and compressive strain occurs in the cortex of the bone where the load is applied. The cortex on the opposite side of the bone will undergo tensile strain as the bone bows away from the load. Initially, small fractures will occur in the cortex undergoing tensile strain because bone is weaker under tension than it is under compression. Once the failure point is reached on the far side from the load, the compressive strain increases markedly and the failure point for the side near the applied load is reached, also, resulting in a complete fracture. This mechanism can be seen in passengers in lateral collisions, pedestrians struck by a passenger car in the tibia and fibula region, or in the upper extremities from direct applied force in victims of assault with a blunt instrument.
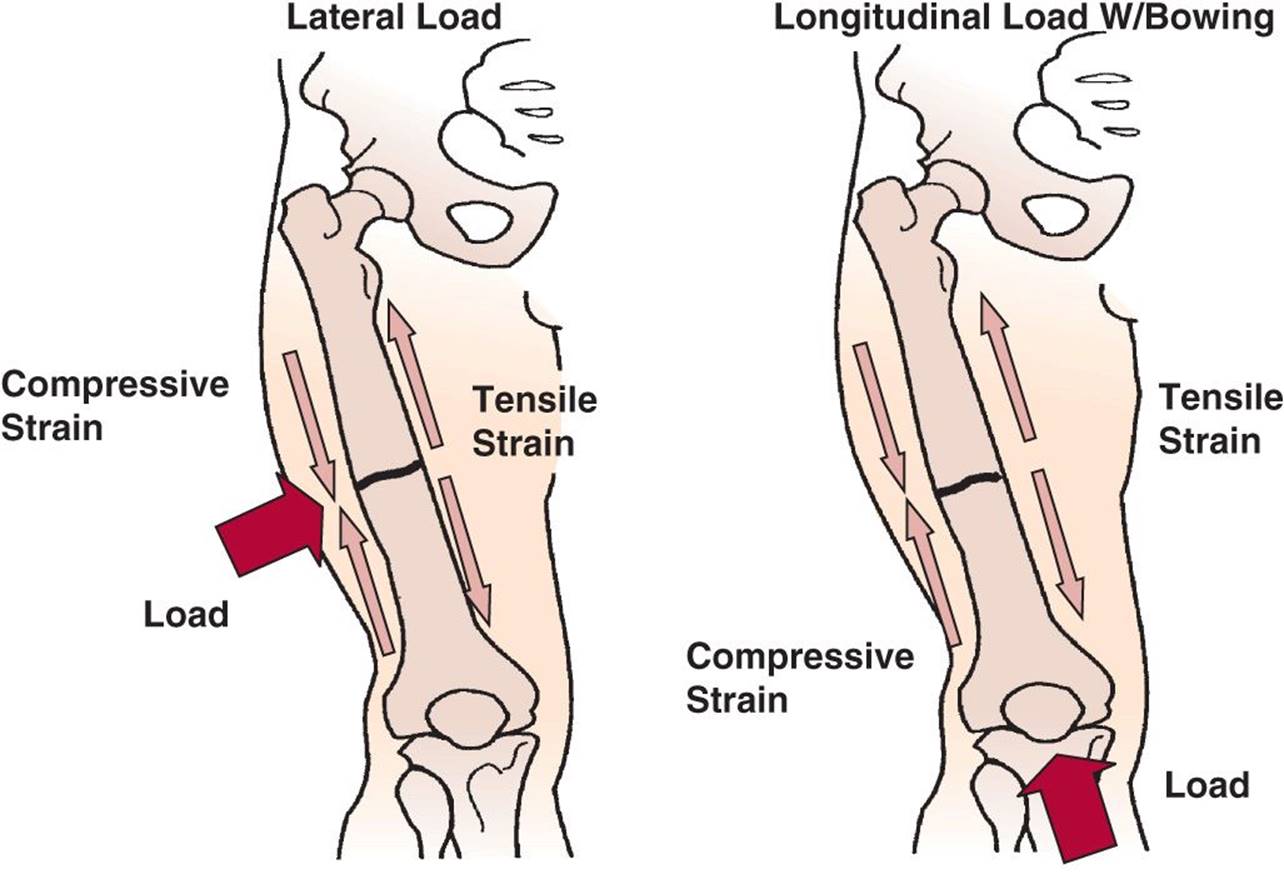

Injury to the Spine and Whiplash
Injury to the vertebral column and spinal cord can be devastating and is frequently the result of a complex combination of specific anatomic features and transmitted forces. These can cause a wide variety of injury patterns distributed through the different portions of the vertebral column. Deceleration forces in motor vehicle crashes, such as impact with the windshield, steering assembly, and instrument panel, inertial differences in the head and torso, or ejection are responsible for both flexion and hyperextension injuries. Although the biomechanics of transmission of force can be readily demonstrated for the vertebral column’s individual components (disks, vertebrae, etc.), a model demonstrating injury patterns in the intact spinal unit is lacking.36 The cervical spine is most frequently injured in motor vehicle crashes, due to its relatively unprotected position compared to the thoracic and lumbar regions. Injuries are related to flexion, extension, or lateral rotation, along with tension or compression forces generated during impact of the head. The direction and degree of loading with impact account for the different injury patterns in trauma to the cervical spine.29 Approximately 65% of injury is related to flexion–compression, about 30% to extension–compression, and 10% to extension–tension injuries.62 Fracture dislocations of the vertebrae are related to flexion and extension mechanisms, whereas fractures of the facets are related to lateral-bending mechanisms. In contrast to trauma to the cervical spine, injury to the thoracic or lumbar spine is more likely related to compressive mechanisms. The rib cage and sternum likely provide stabilizing forces in motor vehicle crashes and lessen the risk of injury in these regions.
Whiplash refers to a pattern of injury seen often in MVCs with a rear-end impact. The injury is usually a musculoligamentous sprain, but may be combined with injury to cervical nerve roots or the spinal cord. Patients typically experience neck pain and muscle spasm, although an additional spectrum of symptoms has been described.63 The etiology of whiplash probably relates to acceleration and extension injury, with some rotational component in non–rear-impact crashes. Factors related to poor recovery following whiplash injury are a combination of sociodemographic, physical, and psychological, and include female gender, low level of education, high initial neck pain, more severe disability, increased levels of somatization, and sleep difficulties.