Pharmacodynamics
Published (updated: ).
Pharmacodynamics in its simplest form asks, “How do drugs do what they do?” Drugs cannot make the body do anything that the body doesn’t normally do. All they do is change the rate of pre-existing physiological processes. The rate of physiological processes is controlled by the rate of neurotransmission. Drugs can alter physiological function by altering the rate of transmission so that there is increased or decreased activation of receptors that regulate that function. Consciousness, for example, is determined by neurotransmission in the ascending reticular activating system (ARAS). Stimulant drugs such as amphetamine increase alertness and wakefulness by enhancing transmission of specific transmitters, whereas depressant drugs such as alcohol bring on sedation by altering the activity of certain neurotransmitters. Peripherally, heart rate is regulated by the activity of the autonomic nervous system. Drugs can stimulate heart rate by increasing the activation of adrenergic receptors that stimulate cardiac activity, while other drugs alter cholinergic transmission to inhibit cardiac activity. Understanding what a drug does means knowing where and how a drug affects neurotransmission.
Altering Neurotransmission
First, it is necessary to pinpoint exactly what is changing when a drug alters neurotransmission. Across all drugs, the ultimate change is the degree of postsynaptic receptor activation which, in turn, alters the rate of the physiological process. Remember that postsynaptic receptors are the receptors on the receiving end of the synapse. When these receptors are activated, they open or close ion channels in the postsynaptic neuron, giving rise to excitatory postsynaptic potentials (EPSPs) or inhibitory postsynaptic potentials (IPSPs). This makes it more or less likely to trigger an action potential.
Drugs can affect postsynaptic receptors by binding to them directly, but they can also bind to presynaptic receptors to influence neurotransmission by altering how much transmitter is released. We will look at both direct and indirect methods, starting with the former. Presynaptic receptors modulate the production and/or release of the transmitter. These receptors can be classified as one of two types, autoreceptors, and heteroreceptors.
Autoreceptors represent one mechanism whereby neurons self-regulate their level of activity. The presence of autoreceptors answers the question, how do nerve terminals know how much neurotransmitter to release into the synapse? They serve as a negative feedback loop—the amount of neurotransmitter released into the synapse can regulate its own release by activating inhibitory autoreceptors, which tells the neuron to stop releasing the neurotransmitter. Autoreceptors are generally located on the presynaptic neuronal mechanism close to where neurotransmitters are released. On the other hand, heteroreceptors are activated by transmitters released from other neurons that regulate the activity of the presynaptic neuron. Activation of presynaptic heteroreceptors can either increase or decrease the release of neurotransmitters from the presynaptic neuron, thus influencing synaptic transmission. The hetero- prefix means “different”. What distinguishes an autoreceptor from a heteroreceptor is the source of the neurotransmitter that activates the receptor. In some cases, the primary neuron and the other neuron may use the same neurotransmitter. But if it originates from the primary neuron, it acts on presynaptic autoreceptors. Conversely, if it originates from the other neuron, it acts on presynaptic heteroreceptors. Heteroreceptors can be located anywhere on the transmitting neurons, in the dendrites, soma, or axon.
Agonists and Antagonists
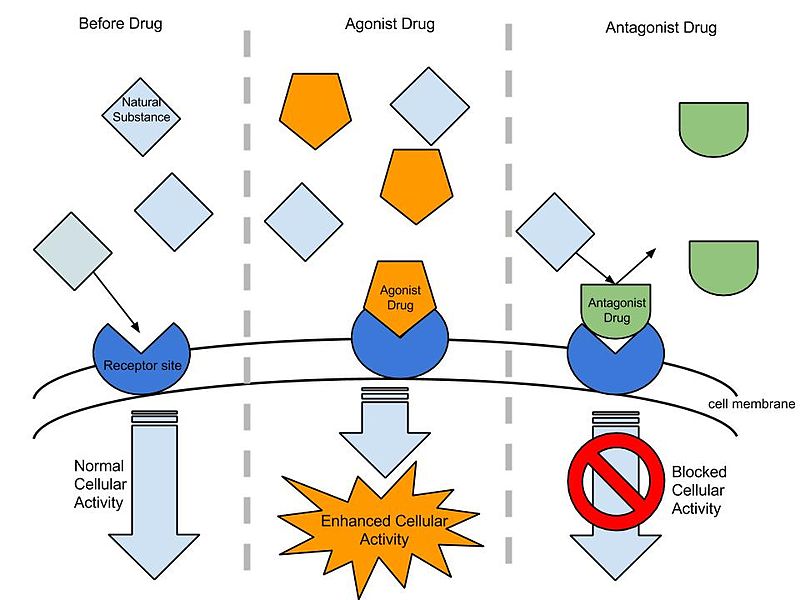
Many receptors have a baseline or basal level of activity. Agonists enhance this, while antagonists keep the activity at or below the baseline, depending on whether there is tonic stimulation of the receptor. However, some drugs can induce exactly the opposite effects mediated by the receptor and are called inverse agonists. Many drugs previously classified as antagonists are being relabeled as inverse agonists because we have learned that they impede the resting activity of receptors. One such example would be those drugs called benzodiazepine inverse agonists because, in contrast to benzodiazepine agonists that cause anxiolytic and anticonvulsant effects, inverse agonists induce anxiety and convulsions.
Indirect Agonists and Antagonists
As we mentioned before, the site of action of a drug need not be limited to the postsynaptic receptor although ultimately the degree of activation of the postsynaptic receptor may be affected. Some drugs can influence neurotransmission at different steps in the process. An indirect agonist is a drug that enhances receptor activity without directly binding to the receptor. One way to accomplish this is to induce the release of a neurotransmitter either directly or activate an excitatory heteroreceptor on the presynaptic neuron. The result would be an enhanced release of transmitters with increased activation of postsynaptic receptors. Amphetamine is such an example of an indirect agonist that is a releasing agent. Releasing agents may or may not possess agonist activity at postsynaptic receptors, but their main effect comes from increased transmitter release.
Another common mechanism of indirect agonism is to increase the amount of neurotransmitter in the synaptic cleft by blocking the mechanisms meant to remove them. The way in which the actions of most neurotransmitters are terminated is through a process called neuronal reuptake. A reuptake inhibitor accomplishes this is by blocking the transporter proteins in the neuronal membrane that are responsible for reclaiming the neurotransmitter from the synaptic cleft. This increases the amount of neurotransmitter available to activate postsynaptic receptors (see image below). Cocaine was the first drug found to block transporter proteins and prevent neuronal reuptake of transmitters.
Reuptake inhibitors are a common type of drug and can be found in many therapeutic medications, such as selective serotonin reuptake inhibitors (SSRIs) or serotonin- norepinephrine reuptake inhibitors (SNRIs).
If the neurotransmitter is normally broken down by enzymes within the synaptic cleft or after reuptake, an enzyme inhibitor can interfere with this process by inhibiting the enzymes responsible. The result is similar to reuptake inhibitors; by preventing the breakdown of the released neurotransmitter, the amount available to activate receptors increases. An example is the monoamine oxidase inhibitor (MAOI) used to treat some forms of depression by preventing monoamine oxidase (MAO) from breaking down monoamine neurotransmitters and increasing the amount of transmitter in the synapse.
It is also possible to have indirect antagonistic effects. By inhibiting the release of neurotransmitters, a drug can indirectly reduce overall receptor activity by decreasing the quantity of neurotransmitter in the synapse. A presynaptic regulator binds to the autoreceptors on the presynaptic neuron and inhibits neurotransmitter release.
Drug Interactions
The effects of a drug can also be influenced by the presence of other drugs or substances. Drug interactions can result in the enhancement or reduction of a drug’s effect. When two drugs with similar effects are used together, the result can be additive, meaning the total response is what you would expect if you added the independent effects of the drugs together. An example is the synergistic effect of multiple drugs that depress neuronal activity such as ethanol plus a sedative drug. Potentiation occurs when one drug has minimal effect by itself but enhances the effects of a second drug. Finally, if a drug reduces the effectiveness of another, this is considered antagonism.