Types of Burns
Published (updated: ).
Thermal
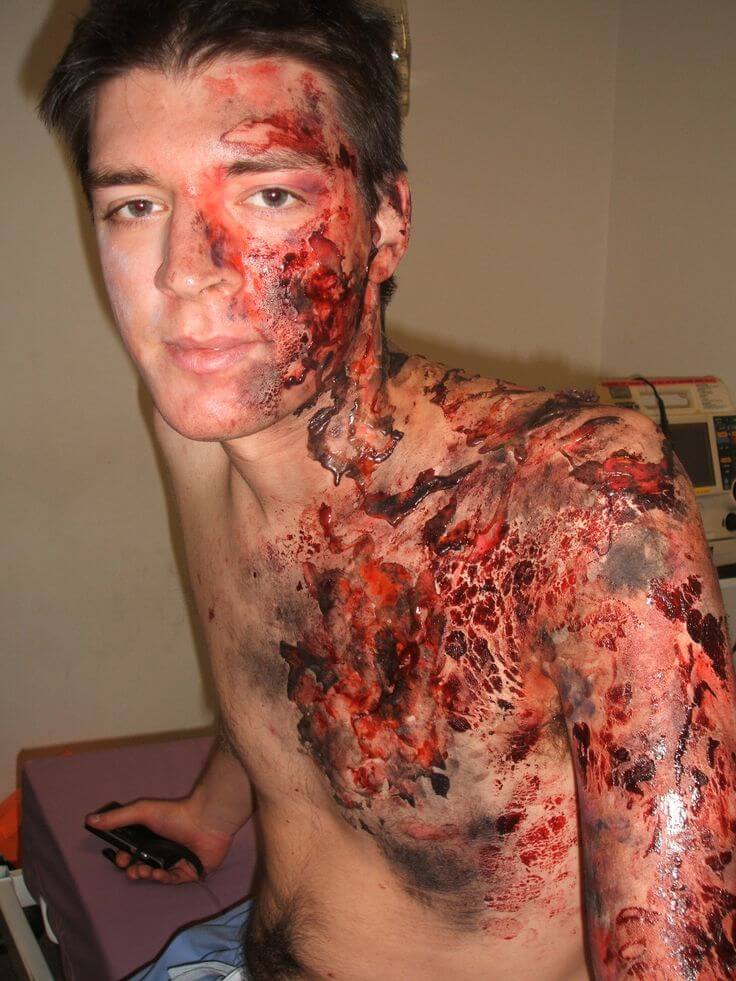
Thermal burns cause both local injuries and, if severe (> 20% of body surface area), a systemic response. The local injuries can be roughly separated into three zones of injury analogous to a circular target pattern. The innermost injury is the zone of coagulation or necrosis, representing the area of irreversible cell death. Surrounding this is the zone of ischemia or stasis, representing an area of decreased circulation and an area at increased risk of progression to necrosis due to hypoperfusion or infection. The outermost area is the zone of hyperemia, representing an area of reversible vasodilation and an area that usually returns to normal. In clinical practice, burns are dynamic injuries that may progress over hours to days, making it difficult to accurately determine the various zones during the early course of the injury.
Large burns (>20% body surface area) also cause a systemic response from the release of inflammatory and vasoactive mediators. Fluid loss locally at the burn site, fluid shifts systemically, plus decreased cardiac output and increased vascular resistance, can all lead to marked hypovolemia and hypoperfusion called “burn shock.”
Inhalation
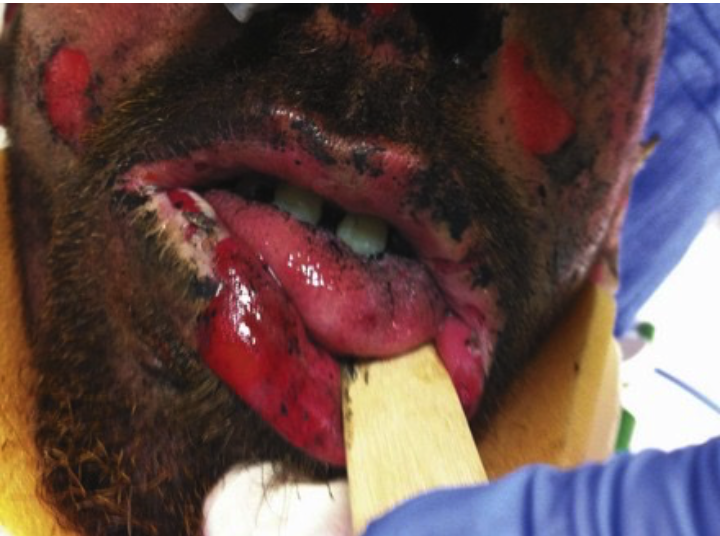
Annually, approximately 3400 patients die from burns or related complications such as smoke inhalation, carbon monoxide or cyanide poisoning, organ failure, or infection. Burn treatment begins at the site of injury. EMS should assess for inhalation injury by looking for singed nasal hairs, burns on the nasal and mouth area, respiratory distress, and sooty sputum. Inhalation injury must be ruled out in the ED. Inhalation injury can lead to upper airway edema within 12 to 24 hours, and the recommendation is for intubation if there is any doubt. Fiberoptic bronchoscopy if possible, can provide an accurate way to determine inhalation injury.
Inhalation injury is a broad term that includes pulmonary exposure to a wide range of chemicals in various forms including smoke, gases, vapors, or fumes. Inhalation injury from smoke exposure is commonly seen in patients exposed to fires. Smoke inhalation is one of the most commonly encountered inhalation injuries.
Smoke inhalation injuries occur when a patient’s respiratory system is exposed to direct heat from fire as well as toxic chemicals that are formed from the decomposition of materials during combustion. The composition of smoke varies with each fire depending upon the materials being burned, the amount of oxygen available to the fire, and the nature of the fire. High-oxygen and high-temperature fires often do not produce large amounts of smoke. Low oxygen fires are often lower temperature fires, and these lower temperatures often give rise to more toxic chemicals, such as carbon monoxide. Other common toxic compounds created in smoke are ammonia, carbon dioxide, hydrogen cyanide, aldehydes, sulfur dioxides, and nitrogen dioxide. These different elements give rise to a combination of gases, airborne solids, and liquid vapors that mix with the ambient air to create smoke. Inhalation of these components, when exposed to smoke, causes both upper and lower airway injury.
According to FEMA records, in 2015, there were 380,940 residential fires, resulting in 2565 deaths, and 11,475 fire-related injuries in the United States. The number of deaths has not decreased since 2006 and has trended 2% upward despite a decrease of 9% in fire injuries during this same period. The leading cause of death from fire injuries remains respiratory failure, and smoke inhalation injuries affect one-third of all burn injury victims.
Inhalation injury affects the respiratory system through damage to the airways (including nasal passages, posterior oropharynx, larynx, trachea, bronchi) or parenchymal damage (alveoli). The location where damage occurs is complex. Thermal injury often affects only to the level of the larynx. Chemical toxin/irritants may cause damage to just the airways, just the alveoli, or both. Specifically, water solubility for gases or vapors, and the physical characteristics of the particulates for fumes and aerosols are important for determining the location of the injury. More water-soluble chemicals will often damage the moist mucosa of the upper airway without causing alveolar damage. Examples of highly water-soluble chemicals include ammonia and sulfur dioxide. Chemical toxins that have low water solubility may reach the lung parenchyma without damage to the airways.
Damage to airway tissue causes increased mucus production, edema, denudation of epithelium, and mucosal ulceration and hemorrhage. Obstruction of airflow is often the effect caused by tissue edema narrowing the passageways and mucus/blood/fluid impeding airflow. A direct thermal injury is rare past the vocal cords because even superheated air is quickly cooled by the nasopharynx and oropharynx prior to causing lower respiratory tract injury. Most lower respiratory tract injury is from smoke particles and the chemicals that they carry.
Chemical
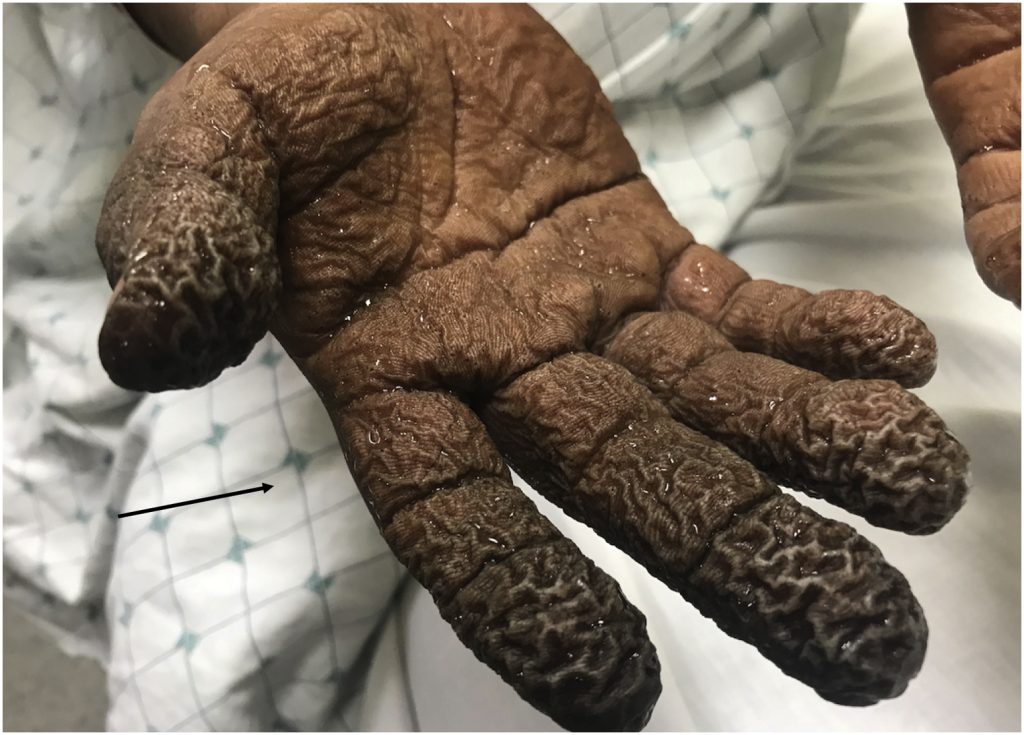
A general approach to the patient with chemical burns involves scene safety, protecting health care workers from exposure, removing the patient from exposure, removing any necessary clothing and jewelry, and brushing off dry chemicals with a suitable instrument. Dry lime in particular should be brushed off before attempting irrigation, because it contains calcium oxide that reacts with water to form calcium hydroxide, a strong alkali. In contrast to thermal burns, many chemicals will continue to induce injury until removed, so immediate clearance of the offending agent is paramount in the intended treatment plan.
For most chemical burn injuries, copious irrigation with water or saline is the initial treatment. The exceptions to this are elemental metals and possibly phenols. Elemental metals produce exothermic reactions when combined with water, whereas aqueous irrigation of phenols may cause deeper infiltration into tissue. Gentle irrigation of chemical burns under low pressure is essential, because higher pressure irrigation can cause deeper infiltration of the chemical into the skin and place the patient and provider at risk for splatter injury. Moderately warm water is often advised. Irrigation should be started promptly, because started initial treatment in the field has been associated with reduced severity of burn injury and a shorter length of hospitalization. Irrigation should begin with the eyes and face, which prevents further inhalation or ingestion or toxin. Treatment should continue until the pH at the skin surface is neutral, which may take 2 hours or more in the case of alkali burns. Ideally, pH at the skin surface should be measured 10 to 15 minutes after discontinuation of irrigation. Litmus paper, if available, is ideal for this purpose. Neutralizing agents are generally not recommended given the potential for an exothermic reaction to occur between the 2 substances. The delay in obtaining the neutralizing agent will also allow for deeper tissue injury if water is readily available.
Electrical
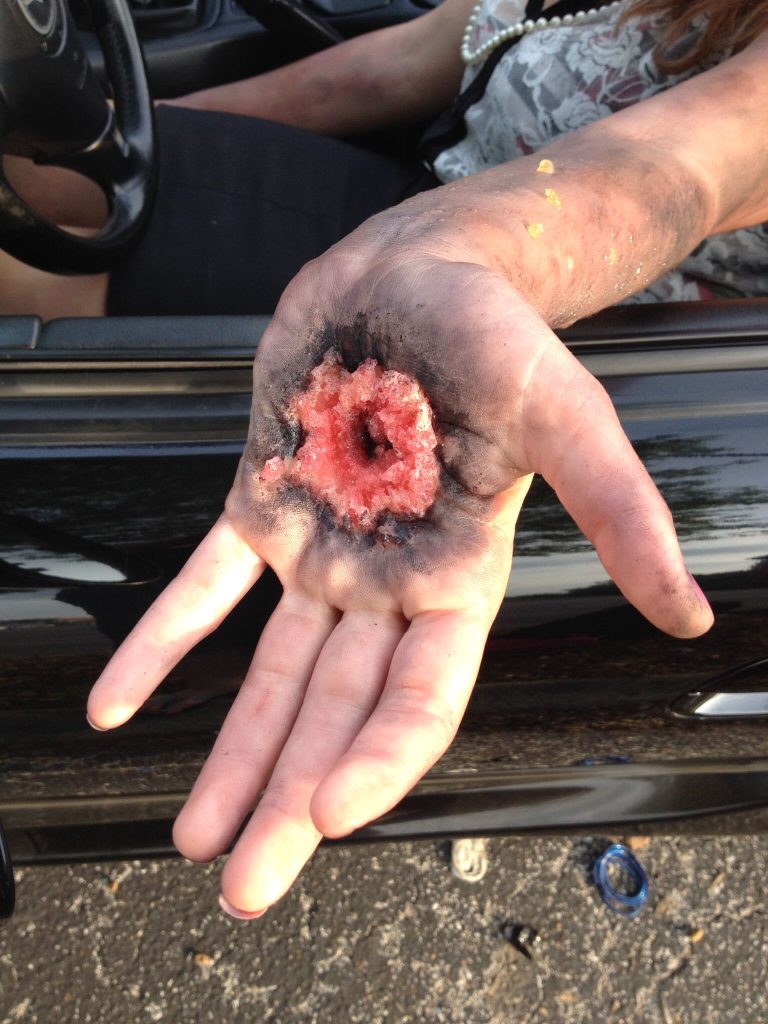
As one of the most devastating and debilitating injuries cared for in burn centers, electrical injuries comprise 4% of all reported causes. Burn surgeons must keep in mind that electrical injuries are unique because they may cause a flash and external burn but also internal burns from the current, which heats up bone and burns muscle as it invests bone. Electrical injuries occur more frequently in adults than children because most result from occupational exposure. Patients who have high-voltage electrical injuries, defined as greater than 1000 V, are at elevated risk of spine fracture injury due to tetany and require complete immobilization until vertebral injury is ruled out. Providers must also evaluate patients with high-voltage injuries for cardiac damage. Direct muscle injury from current flow may cause gross myoglobinuria, requiring more aggressive fluid resuscitation. Patients with gross myoglobinuria often require fasciotomy of affected limbs, and a severe electrical injury often requires monitoring in the intensive care unit. Bone has the highest conductance, and electricity flows along the skeleton, causing significant muscle necrosis adjacent to the bone. TBSA is not necessarily associated with prognosis, and TBSA does not quantify damage to deep tissues in electrical injuries. Entrance and exit wound should be assessed when evaluating which extremities should be closely monitored for compartment syndrome.
Thermal injuries occur as electricity can generate temperatures greater than 100°C. Electroporation occurs as electrical force drives water into lipid membrane, causing cell rupture. Tissue resistance in decreasing order includes bone, fat, tendon, skin, muscle, vessel, nerve. Bone heats to a high temperature and burns surrounding structures, such as muscle, which is the reason muscle swelling and compartment syndrome are common in high-voltage electrical injuries.
Alternating current causes tetanic muscle contraction and the “no let-go” phenomenon. This phenomenon occurs because of simultaneous contraction of (stronger) forearm flexors and (weaker) forearm extensors. Current flow through tissue can cause burns at entrance/exit wounds and hidden injury to deep tissues. Current will preferentially travel along low-resistance pathways. Current will pass through soft tissue, contact high-resistance bone, and travel along bone until it exits to the ground. Vascular injury to nutrient arteries and damage to intima and media can result in thrombosis.
Electrical exposure can cause significant injuries to other organ systems besides the skin and musculoskeletal system. From a cardiac standpoint, arrhythmias are common at the scene (any voltage) or in the hospital (high voltage ≥1000). Heart rhythm should be monitored continuously for at least 24 hours if cardiac injury is suspected at the scene or if a high-voltage injury has occurred. Ventricular fibrillation and asystole are the most common, and Advanced Cardiac Life Support should be instituted immediately. Coronary artery spasm and myocardial injury and infarction have also been described. A normal cardiac rhythm on admission, however, means dysrhythmia is unlikely, and thus, 24-hour monitoring is not needed. In addition, injury to solid organs, acute bowel perforation, and gallstones after myoglobinuria have been described and should be monitored. Myoglobinuria occurs due to the disruption of muscle cells. Myoglobinuria from other causes requires increased fluid administration; however, burn resuscitation usually provides adequate fluid. Cataracts are also a long-term adverse effect of electrical injury, necessitating an ophthalmology evaluation and follow-up.
When taking the patient to the operating room for debridement and grafting of electrical injuries, the physician should perform serial debridements and allow the tissue to completely declare itself. These injuries will often evolve with progressive muscle necrosis over time, thus early grafting (within the first week) often fails to fully close the burn wound. These injuries have similarities to crush injuries, and thus, multiple trips to the operating room for debridement should not be viewed as a failure.
Electrical burns have the potential for 3 types of injury: (1) True electrical injury by current flow; (2) Arc injury from the electrical arc as it passes from the source to an object; and (3) Flame injury from ignition of clothing or surroundings. Electricity arcs occur at temperatures up to 4000°C and can create a slash injury as seen in electricians. Clinicians must keep in mind that the force of the electrical injury may throw the patient, causing additional trauma, ruptured eardrums, and internal organ contusion. Electricity flows through the tissue of greatest resistance, which in humans is bone. In general, the severity of the injury is inversely proportional to the cross-sectional area of the body part with the most severe regions seen in the wrist and ankle and decreasing proximally.
Radiation
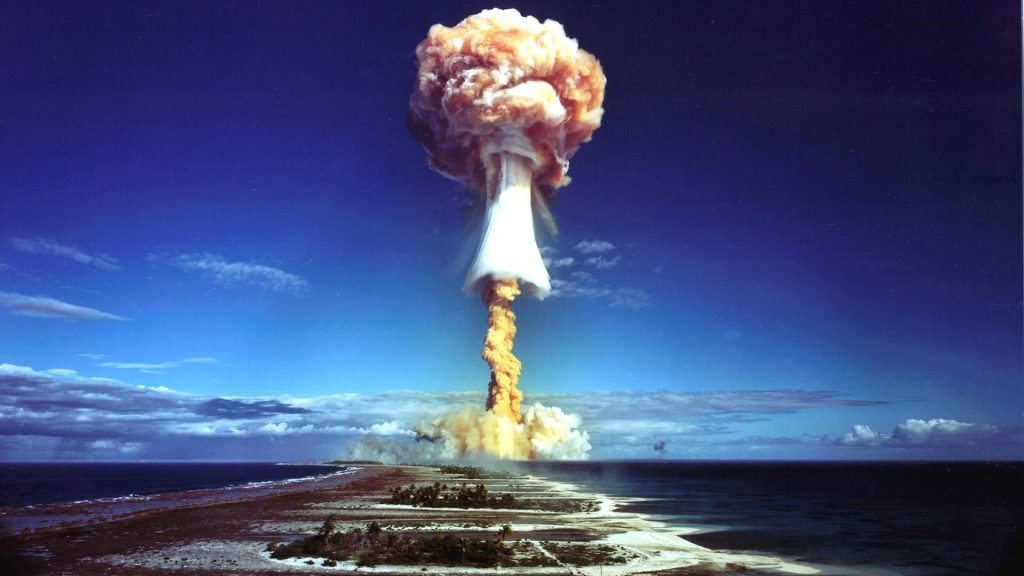
Ever since the world was introduced to the power of nuclear weapons in 1945 with the bombings of Hiroshima and Nagasaki, the world has been forever changed. The power of nuclear weapons was seen firsthand, and the impact of radiation and its impact for injury began to be appreciated. From the bombings of Hiroshima and Nagasaki, many important lessons were learned. One was that proximity to the detonation directly impacted mortality with 86% fatality rates at 0.6 miles from ground zero, and it decreased to 27% at 0.6 to 1.6 miles, and then 2% for those 1.6 to 3.1 miles away. In addition, the mortality was highest during the first 20 days. Of the 122,338 fatalities at Hiroshima, 68,000 occurred within that timeframe. Of the 197,743 survivors, 79,130 were injured, and the remaining 118,613 were uninjured. It is estimated that those injured at Hiroshima contained 90% with burns, 83% with traumatic injuries, and 37% with radiation injuries.
The devastation of these weapons is massive and has some understood properties. The explosion generates high-speed winds that can travel at dramatic speeds. A 20-kiloton nuclear device generates 180 mph winds 0.8 miles from the epicenter. These winds occur with a direct pressure and indirect wind drag, and the pressure wave can destroy windows and buildings and injure parts of the body that are sensitive to pressure changes, such as the lungs and ears. It results in ruptured tympanic membranes, pulmonary contusions, pneumothoraces, and hemothoraces. A fireball that results from the explosion results in thermal injuries and also sends radioactive material into the air. Near ground zero, the thermal injuries are nearly 100% fatal due to incineration from the high temperatures. Radiation is dispersed in a linear fashion and results in burns that vary in severity depending on the distance from ground zero and the time of exposure. Radiation is also dispersed into the air and follows wind patterns, ultimately settling to the ground.
Radioactive material results in both acute injury from immediate exposure and more prolonged injury from delayed exposure to radioactive fallout or contamination. After initial evaluation and decontamination by removing clothing and washing radiation material from the skin, a useful way to estimate exposure is by determining the time to emesis. Patients that do not experience emesis within 4 hours of exposure are unlikely to have severe clinical effects. Emesis within 2 hours suggests a dose of at least 3 Sv, and within 1 hour is at least 4 Sv. The hematologic system follows a similar dose-dependent temporal pattern for predicting radiation exposure, mortality, and treatment.
The combination of radiation exposure to burn wounds has the potential to increase mortality compared with traditional burns. Early closure of wounds before radiation depletes circulating lymphocytes may be needed for wound healing (which occurs within 48 hours). Also, in radiation injuries combined with burn or trauma, laboratory lymphocyte counts may be unreliable. A significant difference between burn/traumatic injuries and radiation injuries is that burn/traumatic injuries can result in higher mortality when not treated within hours.
Decontamination and triage are vital to maximize the number of survivors. Initial decontamination requires removal of clothing and washing wounds with water. Irrigation fluid should be collected to prevent radiation spread into the water supply. Work by many professional organizations, including the American Burn Association, has focused on nationwide triage for disasters and will be vital to save as many lives as possible. Partnerships between emergency medical services, emergency medicine, trauma surgeons, burn surgeons, medical oncology, radiation oncology, and others will be vital because their injuries will require multidisciplinary care in ways not experienced before in modern medicine. It is quite possible that expectant or comfort care could be offered to more patients than typically seen in civilian hospitals, because of resource availability after the disaster.
EMS and the first 24 hours of a burn
The incipient phase of a burn occurs immediately upon contact with the burn. The patient will experience tremendous pain, but no drop in blood pressure. The patient needs narcotics and maybe some fluids. What the patient needs most is to actually be transported to the correct hospital. The incipient phase of a burn lasts for 8 hours.
The second phase of a serious burn injury is the fluid shift phase. The larger the burn, the greater the systemic shift of fluids from the vascular space to the intracellular (third) space. Large burns (>20% body surface area) also cause a systemic response from the release of inflammatory and vasoactive mediators. Fluid loss locally at the burn site, fluid shifts systemically, plus decreased cardiac output and increased vascular resistance, can all lead to marked hypovolemia and hypoperfusion called “burn shock.” The ‘burn shock’ is not only a concern for the patient’s hemodynamic stability, but also for the integrity of the patient’s skin. The fluid will shift to skin and result in massive swelling. The swelling combined with the initial injury of the burn can result in tearing of the patient’s flesh. The damage from the swelling seen in the second phase of a burn can result in irreparable damage to the patient’s skin. The second phase of the burn lasts between 8 and 24 hours.
After the patient has survived the first 24 hours of the burn, the body begins to repair the massive damage to the skin. This recovery leads the patient to consume tens of thousands of calories per day. The patient typically requires parenteral nutrition to meet the nutritional demands of the body healing from a massive burn.