Structures Of The Heart
Published .
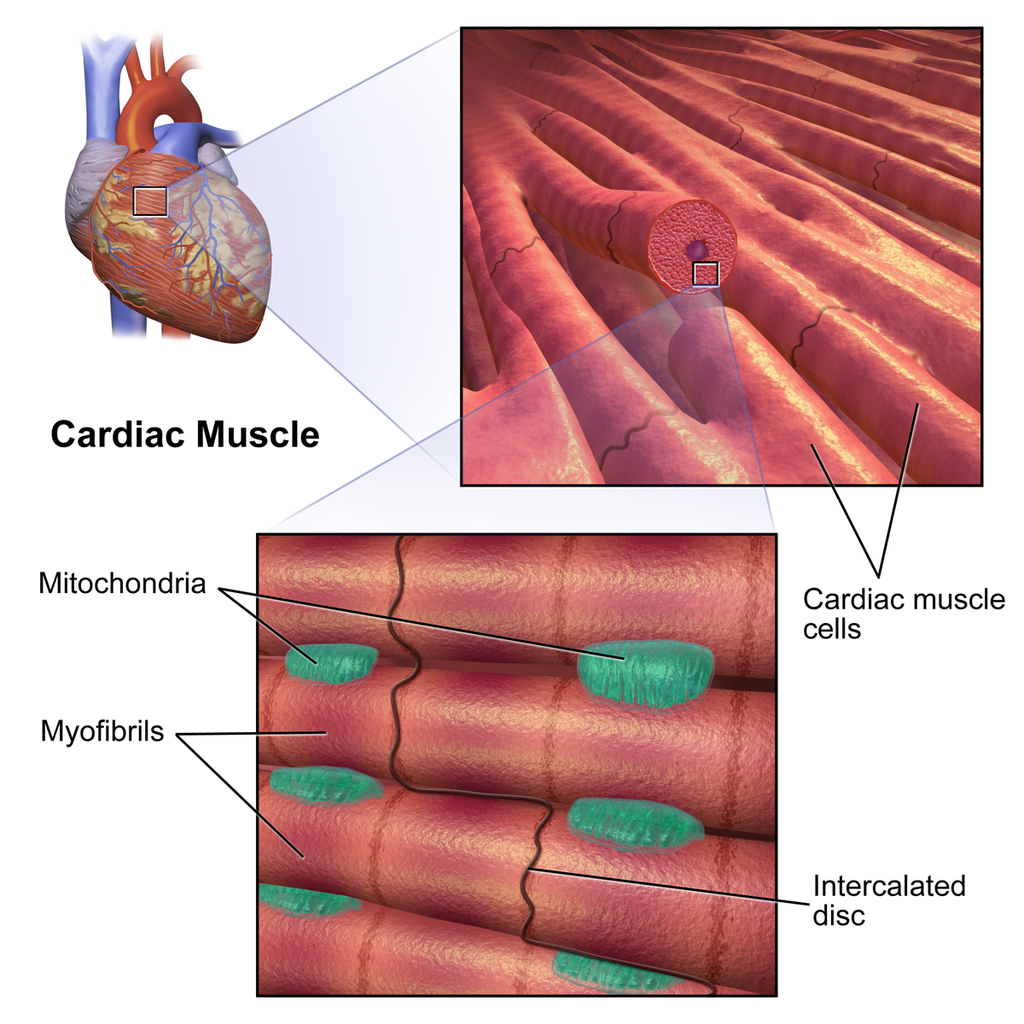
Cardiac Muscle Contracts, Conducts Electricity, And Sometimes Generates It’s Own Impulse
Recall that cardiac muscle shares a few characteristics with both skeletal muscle and smooth muscle, but it has some unique properties of its own. Not the least of these exceptional properties is its ability to initiate an electrical potential at a fixed rate that spreads rapidly from cell to cell to trigger the contractile mechanism. This property is known as autorhythmicity. Neither smooth nor skeletal muscle can do this. Even though cardiac muscle has autorhythmicity, heart rate is modulated by the endocrine and nervous systems.
There are two major types of cardiac muscle cells: myocardial contractile cells and myocardial conducting cells. The myocardial contractile cells constitute the bulk (99 percent) of the cells in the atria and ventricles. Contractile cells conduct impulses and are responsible for contractions that pump blood through the body. The myocardial conducting cells (1 percent of the cells) form the conduction system of the heart. Except for Purkinje cells, they are generally much smaller than the contractile cells and have few of the myofibrils or filaments needed for contraction. Their function is similar in many respects to neurons, although they are specialized muscle cells. Myocardial conduction cells initiate and propagate the action potential (the electrical impulse) that travels throughout the heart and triggers the contractions that propel the blood.
Cardiac muscle undergoes aerobic respiration patterns, primarily metabolizing lipids and carbohydrates. Myoglobin, lipids, and glycogen are all stored within the cytoplasm. Cardiac muscle cells undergo twitch-type contractions with long refractory periods followed by brief relaxation periods. The relaxation is essential so the heart can fill with blood for the next cycle. The refractory period is very long to prevent the possibility of tetany, a condition in which muscle remains involuntarily contracted. In the heart, tetany is not compatible with life, since it would prevent the heart from pumping blood.
Control of Heart Rate
In the intrinsic conduction system, heartbeats originate from the rhythmic pacing discharge from the sinoatrial node (SA node) within the heart itself. The SA node, located in the right atrium, is a part of the intrinsic conduction (or nervous) system found in the heart. This conduction system in order of rate of depolarization starts with the SA node or pacemaker and results in atrial depolarization and atrial contraction, the internodal pathway, the AV node (where the impulse is delayed), AV bundle, the left and right branches of the bundle of His and lastly the Purkinje fibers, both of which result in ventricular depolarization and contraction. All of the components of the intrinsic conduction system contain autorhythmic cells that spontaneously depolarize. In the absence of extrinsic neural or hormonal influences, the SA node pacing rate would be about 100 beats per minute (bpm). The heart rate and cardiac output, however, must vary in response to the needs of the body’s cells for oxygen and nutrients under varying conditions. In order to respond rapidly to changing requirements of the body’s tissues, the heart rate and contractility are regulated by the autonomic nervous system, hormones, and other factors.
The Battle Of The Autonomic Nervous System
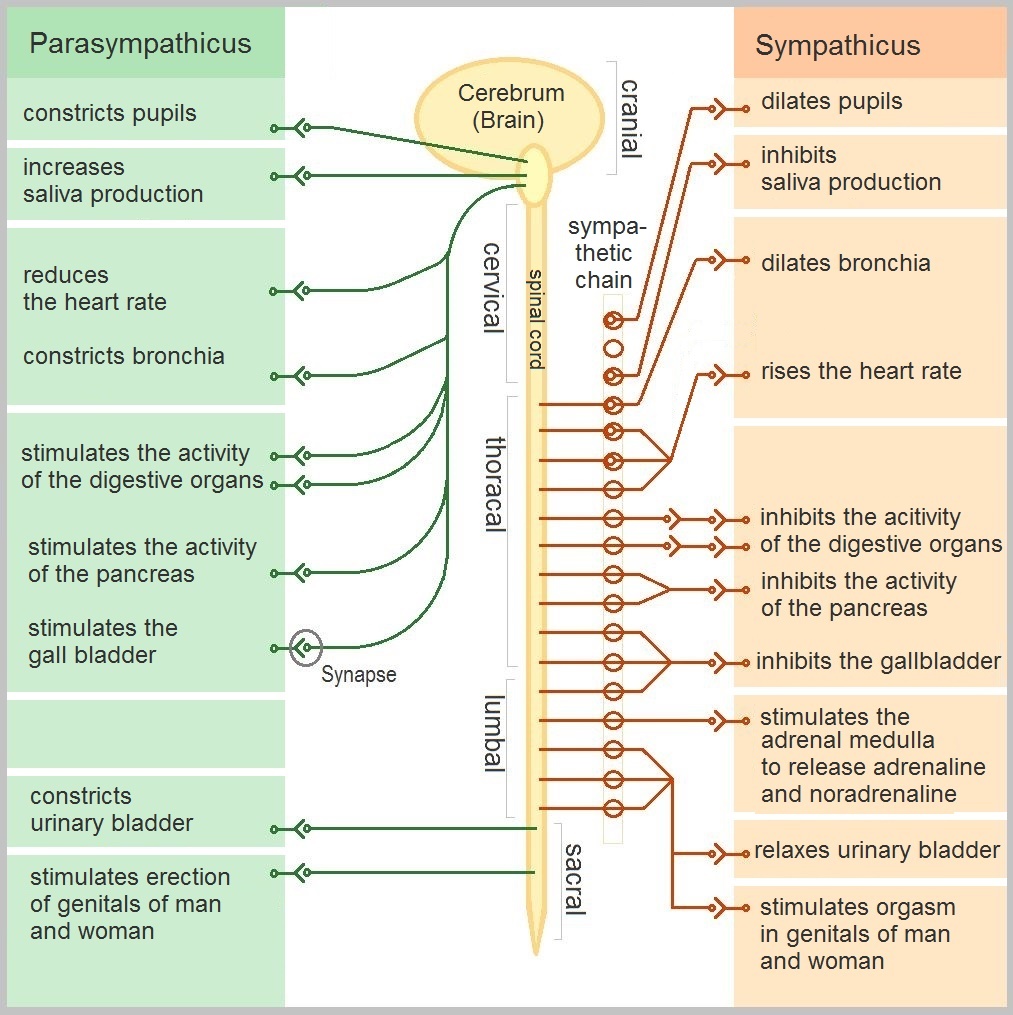
The autonomic nervous system (ANS) is the component of the peripheral nervous system that controls cardiac muscle contraction, visceral activities, and glandular functions of the body. Specifically the ANS can regulate heart rate, blood pressure, rate of respiration, body temperature, sweating, gastrointestinal motility and secretion, as well as other visceral activities that maintain homeostasis[1–4]. The ANS functions continuously without conscious effort. The ANS, however, is controlled by centers located in the spinal cord, brain stem, and hypothalamus.
The ANS has two interacting systems: the sympathetic and parasympathetic systems. The sympathetic and parasympathetic neurons exert antagonistic effects on the heart. The sympathetic system prepares the body for energy expenditure, emergency or stressful situations, i.e., fight or flight. Conversely, the parasympathetic system is most active under restful conditions. The parasympathetic counteracts the sympathetic system after a stressful event and restores the body to a restful state. The sympathetic nervous system releases norepinephrine (NE) while the parasympathetic nervous system releases acetylcholine (ACh). Sympathetic stimulation increases heart rate and myocardial contractility. During exercise, emotional excitement, or under various pathological conditions (e.g., heart failure), the sympathetic nervous system is activated. The stimulation of the sympathetic nervous system causes pupil dilatation, bronchiole dilatation, blood vessel constriction, sweat secretion, inhibits peristalsis, increases renin secretion by the kidneys, as well as can induce reproductive organ contraction and secretion. In contrast, parasympathetic stimulation decreases heart rate and constricts the pupils. It also increases secretion of the eye glands, increases peristalsis, increases secretion of salivary and pancreatic glands, and constricts bronchioles. Most organs receive innervations from both systems, which usually exert opposing actions. However, this is not always the case. Some systems do not have a response to parasympathetic stimulation. For example, most blood vessels lack parasympathetic innervations and their diameter is regulated by sympathetic nervous system input, so that they have a constant state of sympathetic tone. It is a decrease in sympathetic stimulation or tone that allows vasodilatation. During rest, sleep, or emotional tranquility, the parasympathetic nervous system predominates and controls the heart rate at a resting rate of 60-75 bpm. At any given time, the effect of the ANS on the heart is the net balance between the opposing actions of the sympathetic and parasympathetic systems.